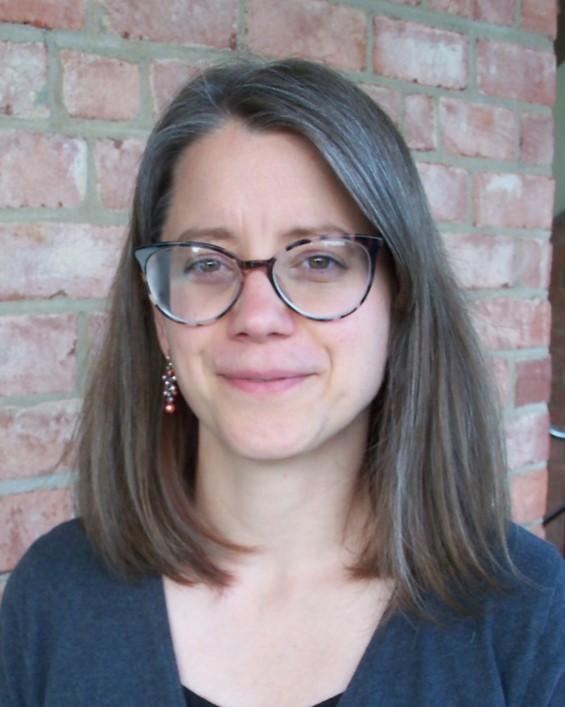
When joining the University of Kentucky physics department as a graduate student in 2006, I knew that I enjoyed doing experimental research but had not yet settled on a research topic. With the wide variety of research topics being pursued at UK, it was an ideal place to spend my first two semesters learning more before starting my research in the summer of 2007 in Dr. Joe Brill’s lab. What caught my interest was electrical conduction through organic molecular materials, which was the topic of my research for my Ph.D. and which I continue to study as a physicist at the National Institute of Standards and Technology (NIST) in Gaithersburg, MD.
The charge and energy carriers in organic semiconductors act differently than standard semiconductors, such as Si, but can still be used to make electronic devices such as transistors and LEDs. In organic molecular semiconductors, weak bonding between neighboring molecules creates an environment where charges are more likely to “hop” between sites to get across the material, whereas in Si, charge carriers will move through energetic bands that are like roadways. This hopping slows down the charge movement, but the same weak bonding lends mechanical flexibility and can be used to isolate interesting excited states within a matrix of molecules. In addition, the unique atomic and dielectric environment in organic materials lead to intrinsically long spin lifetimes and unique excitons states. These interesting properties are inspiring new research using organic materials in memory devices and in future computing paradigms, such as neuromorphic and quantum computing.
After graduating in 2013, I joined NIST as a post doc and studied organic semiconductor transistors. Measurements of total device function of a transistor, LED, or other electronic device is influenced by both the device and material physics. This intermixing of phenomena within a device makes isolating one portion for further study very difficult, but understanding each component is important for improving the performance of the overall device. During my first few years at NIST, we were able to isolate the effects of contact resistance from the semiconductor material physics showing the overwhelming role that poor charge injection has on a key semiconductor metric measured using transistors.
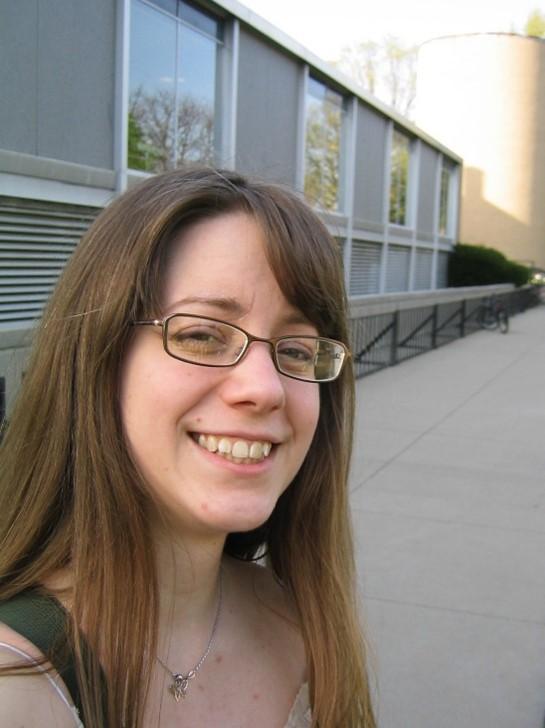
In 2019, I was hired as a permanent staff member at NIST working in the Nanoscale Device Characterization Division. The projects that my research team is working on today focus on new functionality in molecular and polymer materials. Our recent work has used the magnetic field dependence in light-to-charge conversion in molecules as a field sensor that can pick up both magnitude and direction of the magnetic field. Our hope is to push this further into localized measurements and to explore more applications of the unique exciton, spin, and charge physics in organic materials. In conjunction with these efforts, we continue to study the limitations and possibilities in characterization of new semiconducting materials using electronic devices.
The University of Kentucky Department of Physics and Astronomy was instrumental in launching my career and is the home to some of my fondest memories. I now work in an environment surrounded by inspiring scientists and wonderful humans working to create and characterize technologies of the future. The education I received in class and in the lab make my current work possible.